SUPER MASSIVE BLACK HOLES
Poshak Gandhi
European Southern Observatory, Chile
pg@ast.cam.ac.uk
January 2003
Abstract
All large galaxies are now known to contain massive black holes (BHs) at their centres. New X-ray telescopes are finding thousands of powerful BHs emitting large amounts of radiation across the cosmos, and detailed studies suggest that these could influence the formation of the galaxies in which they lie. The aim of this article is to provide a general introduction to recent findings related to these Super-Massive Black Holes at very large distances from Earth, especially from the point-of-view of X-ray observations.
The Big Picture
One of the questions I am constantly asked about my work is "But do you really believe in the existence of black holes??". My answer to this is an emphatic "Yes". Astronomers now have a lot of indirect evidence that black holes exist. True, we do not have any direct evidence yet (for example, an 'image' of a black hole), but that will almost certainly be obtained in the future. Astronomers can now tell you a lot about our Universe with a reasonable amount of confidence. We now know that we live in a Universe which originated in a 'large inflated explosion' approximately 14 billion years. This Big Bang led to the current expansion of the Universe, and this expansion is actually accelerating, i.e. the Universe will continue to grow at an ever-increasing rate, and there is not enough matter in the Universe to halt this growth by gravity.
This remarkable advance in our knowledge has been made possible, in large part, by many technological developments which have allowed us to build larger and better telescopes. Telescopes with visible-light collecting mirrors as large as 10 metres in diameter are now operational, and there are active plans to build 100 metre telescopes. Telescopes orbiting the Earth in space provide images of exquisite quality, since they are above the blurring atmosphere, and also give access to regions of the electromagnetic spectrum not usually visible from Earth - e.g., X-rays, Gamma-rays and long-wavelength infrared radiation.
An important discovery of the past few years has been the fact that all nearby 'large' galaxies contain very compact, gravitating centres or nuclei which can only be massive black holes (see, for example, Kormendy & Richstone 1995). Black holes (BHs) were first predicted to exist by Subrahmanyan Chandrasekhar in 1919 as the remnants of single massive stars after they have exhausted their fusion supply of Hydrogen and other heavy elements. The BHs now being discovered in other galaxies, however, have the same mass as a million or even a billion Suns put-together in a region smaller than the size of our Solar System. In about 10 per cent of galaxies, these BHs are extraordinarily bright and can be seen to very large distances across the cosmos. These systems are called Active Galactic Nuclei (AGN) and are the subject of intense research. They provide the opportunity to study extreme physical conditions not reproducable in a laboratory, and their immense power can influence the formation and evolution of the galaxies which host them. Moreover, we do not understand whether these BHs formed before or after the first stars in the Universe, or whether their growth is concurrent with that of galaxies. Figure 1 shows an image of one such AGN compared to a galaxy with a central BH which is not actively radiating light (Note: there are many lines of evidence which have convinced astronomers of the reality of BHs themselves. For more information, you are referred to the Recommended Reading articles at the end).
Figure 1:
An example of a galaxy hosting a relatively powerful active nucleus (left-hand figure) compared to a galaxy whose central black hole is not active (right-hand figure). The four spikes on the active nucleus are a remnant of the telescope structure seen because the nucleus is a very bright point. (Telescope: HST; Source: Niel Brandt)
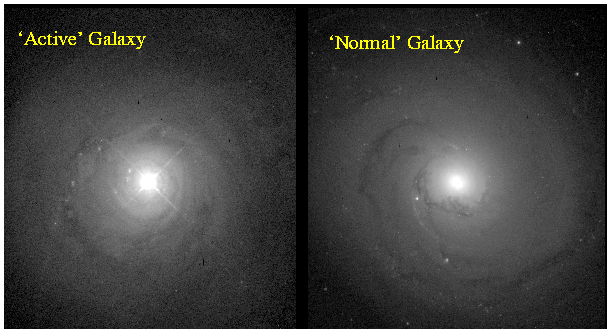 |
Active Galactic Nuclei
A powerful AGN can produce more energy than an entire galaxy containing about a 100 billion stars (in the same amount of time). What is the mechanism which powers such a colossal output? It is now widely accepted to be the release of gravitational potential energy of infalling material on to the black hole. If the Earth were turned into a BH and you could drop a ball so that it fell towards the 'surface', or event horizon, of this BH, the total energy gained by the ball before it is swallowed by the BH would be greater than the energy output of converting every Hydrogen atom in the ball (assume it is made of Hydrogen) to Helium by nuclear fusion, such as happens in stars. In other words, the release of gravitational potential energy onto a compact gravitating object is more efficient than stellar nuclear reactions (from 10 to 50 times more efficient). That is why AGN can be so bright. In summary, BHs themselves do not emit light -- as matter is attracted to the BH due to its intense gravity, it collides with other matter and radiation, thus becoming extremely hot and radiating.
Figure 2 shows a cartoon of how an AGN may look. Infalling matter spirals in towards the centre and settles into an accretion disk, from where it is finally swallowed by the black hole. The radiation emitted in the accretion process can sometimes be ejected in the form of a powerful radio jet (probably depending on the strength of magnetic field coupling between the accretion disk and the black hole; but no one really understands how!). The radiation ionizes most of the surrounding gas, leading to emission of characteristic recombination photons. The existence of a large amount of cold gas and dust clouds distributed in a roughly toroidal geometry surrounding the AGN (the dusty torus) has been proposed from observartions of various types of AGN.
This torus is capable of absorbing a large amount of the light emitted from the AGN. But the radiation which is emitted perpendicular to the plane of the torus will easily escape. Now, the relative angle between the plane of this torus and the line-of-sight to Earth will be completely random for different AGN. Thus, in some AGN (relative angle is closer to zero degrees), this light will be absorbed and the AGN would appear as obscured, while in others (relative angle is closer to 90 degrees), we would see the primary AGN light and classify it as an unobscured AGN. Since we see the black hole 'directly' in unobscured AGN, these are typically brighter than their obscured counterparts. Even though this may be an over-simplification, this dusty torus can thus 'unify' observations of different AGN classifications. The central engine is similar in all AGN, and many observed differences can be naturally explained as due to differences in observing view-points.
While AGN emit radiation across the entire electromagnetic spectrum, it turns out that X-ray observations are one of the best means to find black holes. Whereas optical observations can be dominated by light from stars in a galaxy, X-rays are emitted from the hottest matter very close to the event horizon of a BH.
Figure 2:
Schematic of an AGN. The central Black Hole accretes infalling matter from the Accretion Disk and emits high energy radiation which can ionize gas in the surroundings. Often this radiation is seen in the form of a powerful Jet extending over thousands of light-years. The Dusty Torus can absorb a lot of the black hole radiation. If the orientation of the AGN is such that light reaches Earth directly (without passing through the Torus), we would see an unobscured AGN. Otherwise, an obscured AGN would be observed. In some cases, this obscuration can absorb most of the AGN light. THIS DIAGRAM IS NOT TO SCALE!
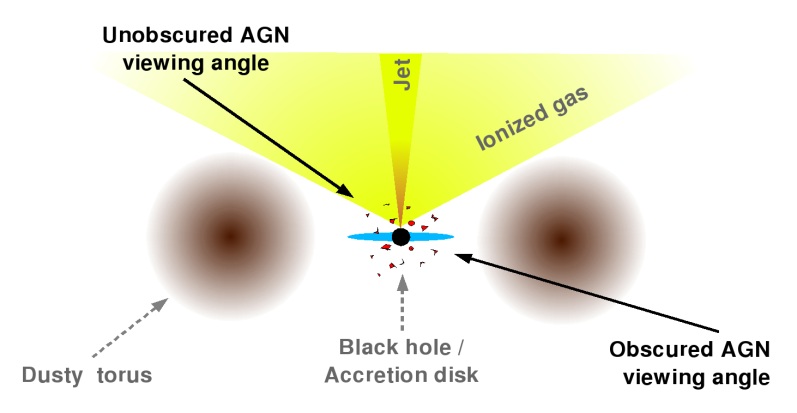 |
Cosmic Background Radiations
So, if every galaxy hosts a central super-massive black hole (SMBH) and if 10 per cent of these are seen to be active today, it is possible that many more were active in the past. If these AGN are then spread across the cosmos, their integrated emission would create a 'glow' of background radiation even if they cannot be distinguished individually. This is exactly what was discovered in 1962, when the first X-ray satellite (essentially consisting of geiger counters) was launched (Giacconi et al. 1962). The aim of that mission was to search for X-ray emission from the Moon (in preparation for the Moon landing); they instead found the Cosmic X-ray Background Radiation (CXB).
This was the first of the cosmic backgrounds to be discovered, even before the better-known Cosmic Microwave Background Radiation, which is now understood to be a remnant of the Big Bang. Such backgrounds are now used as an indispensable tool to study the nature of processes in the distant Universe, which are too faint to be resolved individually. Figure 3 shows a plot of the background energy density in the Universe, as a function of frequency. Completely different processes give rise to, say, the Microwave Background as compared to the InfraRed Background.
Figure 3:
Spectra of various Cosmic Background radiations as a function of frequency (Reproduced from Hasinger 2000). Only the cosmic microwave background (CMB) is thought to be a remnant of the Big Bang; the rest originated in processes much later in cosmic time. For example, the optical background (COB) is due to the combined light of unresolved galaxies. There are almost certainly connections between the various backgrounds. For instance, it is thought that some of the X-ray background (CXB) energy is absorbed and re-radiated as the infrared background (CIB).
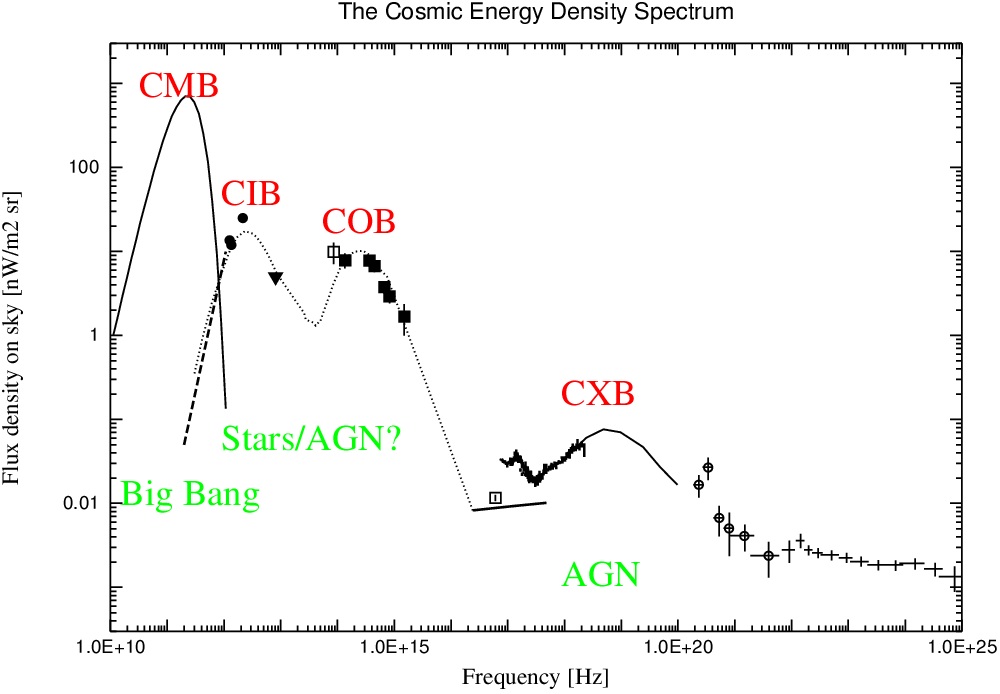 |
X-ray Telescopes
But it took more than 30 years before a conclusive answer to the origin of the X-ray Background was found. This delay was primarily due to the fact that X-ray telescopes are difficult to build and the observations must be carried out from space, since the Earth's atmosphere scatters all such high-energy cosmic radiation via photo-electric absorption and Compton scattering. Moreover, X-ray imaging telescopes typically work by grazing-incidence reflection, i.e., X-ray photons are reflected off mirrors at incidence angles as small as 1 degree. Parallel-incidence reflection (as in all optical reflecting telescopes) would simply absorb X-rays without focusing them. It is a very complicated task to design good grazing-incidence mirrors with a large light-collecting area. The new-generation of X-ray telescopes have been designed to satisfy these conditions. Two of these are currently operational: the Chandra X-ray Observatory and XMM-Newton.
Obscured AGN
While previous X-ray telescopes found a lot of bright AGN (remember that unobscured AGN are typically brighter), they did not possess adequate sensitivity and focusing power to find very distant and faint AGN (the obscured ones). This situation has changed with Chandra and XMM-Newton. Figure 4 shows a very deep of the sky around a region called the Hubble Deep Field-North, which has been well-studied many many optical, infrared and radio telescopes. Shown alongside is also the Chandra X-ray image of this region (this is essentially a photograph with a continuous exposure time of more than 16 days and nights!!). The majority of sources seen in the image are SMBHs (stars can also be detected in X-rays, but they are typically fainter than SMBHs). Note the different colours of the detected sources in X-rays, which have been assigned depending on the individual spectra: red colours signify unobscured AGN. But the existence of different types of black holes has been confirmed with the blue/green coloured black holes. Their spectral shape is characteristic of obscured AGN and matches the spectrum of the CXB itself.
Thus, the sources which produce the CXB have been discovered. All the effort now is concentrated on understanding the obscuration properties of these AGN. There are some puzzles includes the fact that the obscuration inferred from X-ray observations does not always match the scattering inferred from optical light. Moreover, the distribution of these AGN across the cosmos seems to be different from the space distribution of unobscured AGN, and it is possible that they are related to other powerful infrared galaxies which result from mergers of large galaxies. To answer these questions, we will have to await the launch of large telescopes sensitive in the infrared and other wavebands.
Figure 4:
The region of the Hubble Deep Field - North as seen in optical light (right-hand figure). The galaxies which have been circled have also been detected in the X-ray image (left-hand figure) by the Chandra telescope (top right). The numbers on the right hand side denote redshifts, signifying the distances of the galaxies from Earth (Brandt et al. 2002).
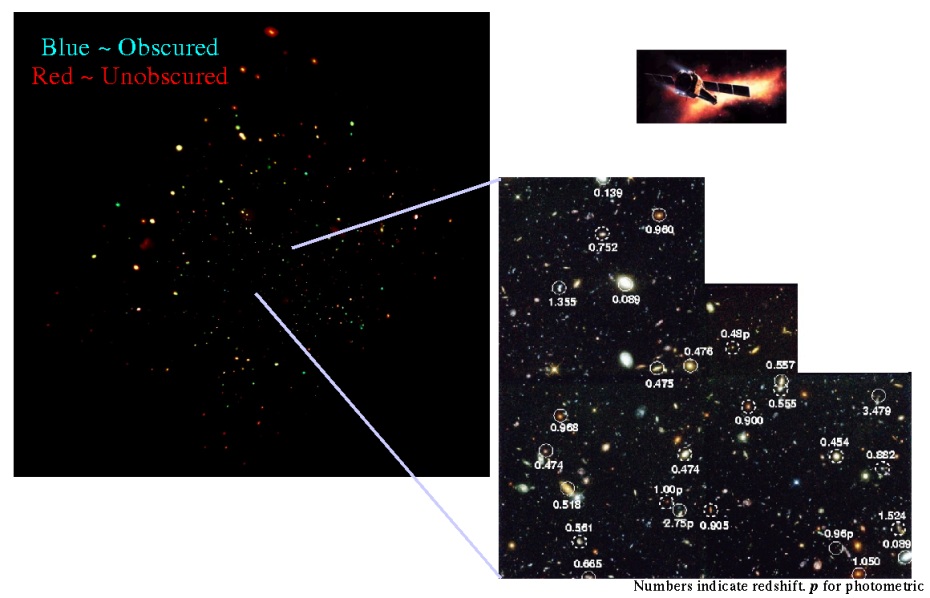 |
Summary
The present era of high energy telescopes, combined with powerful telescopes at other wavelengths, have opened up new eyes on to the Universe. We can now study galaxies in formation and other celestial processes in detail at distances so large that light takes several billions of years to reach us, and the Universe is much younger at these epochs. Black holes have been enigmatic objects for a long time, but X-ray observations give us the opportunity to study their immediate environments. It seems that supermassive black holes significantly affect the formation and evolution of galaxies, though the details are unclear at present. Even our Milky Way galaxy has a black hole at its centre with a mass of approximately 3 million Suns whose origin and evolution we would like to understand. Young, budding astronomers like YOU are needed to solve many of the current puzzles!
References
(May need to use Ghostview or Acrobat Reader to read)
- Brandt W.N. et al., 2002, The Chandra Deep Field-North Survey and the Cosmic X-ray Background, Philosophical Transactions of the Royal Society, Series A
- Giacconi R. et al., 1962, Evidence for x Rays From Sources Outside the Solar System, Physical Review Letters, 1962, 9, p. 439
- Hasinger G., 2000, The sources of the X-ray background, Symposium 'New Visions of the X-ray Universe in the XMM-Newton and Chandra Era', 26-30. November 2001, ESTEC, The Netherlands
- Kormendy J., Richstone D., 1995, Inward Bound: The search for supermassive black holes in galaxy nuclei, Annual Reviews of Astronomy ;& Astrophysics, 33, p. 581
Recommended Reading / Browsing
(Ask College to acquire the books!)
- Gravity's Fatal Attraction, M. Begelman, M.J. Rees, W H Freeman & Co; ISBN: 0716760290; April 1998
- An Introduction to Active Galactic Nuclei, B.M. Peterson, Cambridge Univ Pr; 1997
- Black Holes and Time Warps: Einstein's Outrageous Legacy, K.S. Thorne, W.W. Norton & Company; ISBN: 0393312763; reprint 1995
- The Lighter Side of Gravity, J.V. Narlikar, Cambridge Univ Pr; ISBN: 0521565650; 2nd edition 1996
- Active Galactic Nuclei, J.H. Krolik, Princeton Univ Pr; ISBN: 0691011516; 1998
- The official Chandra X-ray Observatory website
- Gallery of AGN images
- Astronomy Picture of the Day (updated each day)
- New Astronomy Publications (updated each day)